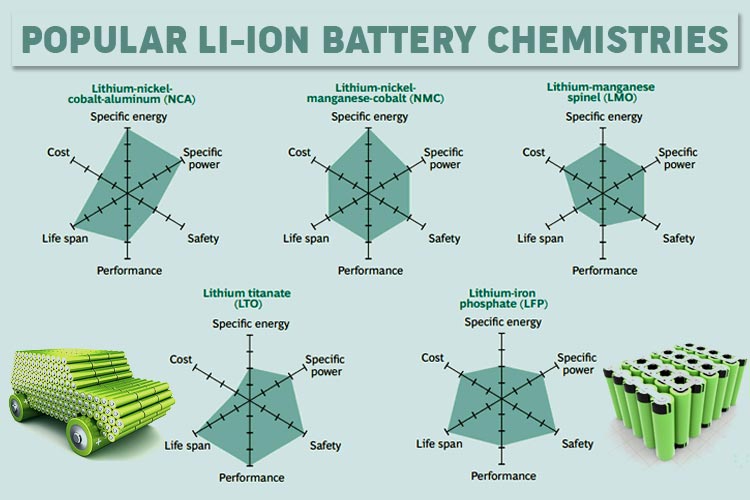
Cells have been used for storing energy from time immemorial, from a Baghdad battery, which dates back to 250BC to newer technology such as a hydrogen fuel cell, that just gives water as a byproduct, batteries have come a long way. A group of cells when connected together is called a battery.
Batteries are touted as the future of energy storage for Electric Vehicles. Even the first cars, made in the year 1842 were powered by batteries, which is almost 2 decades before the invention of Internal combustion engine vehicles but the lack of range and slow charge rate caused the decline of Electric Vehicles in the 20th century. The growing environmental concern due to climate change has forced us to move towards renewable energy and adopt battery energy storage systems and the research and development of battery technologies kick-started.
In a country like India, more than a quarter of total pollution is caused due to automobiles. Till date the adoption of Electric Vehicles (EV) could not take off due to range anxiety, price, lack of infrastructure, and lack of choices. The research and development in Li-ion batteries in recent years have paved the way for EVs across the globe. We have previously discussed the basics of electric vehicle batteries in general. In this article we will be learning about different Li-ion chemistries, their advantages and disadvantages and compare them with few other rechargeable batteries. You can also check out the article on different types of batteries if you want to learn more about batteries in general.
Primary Cell and Secondary Cell
Primary cells are single-use, non-rechargeable cells, the ones we use in our TV remotes, wall clocks, etc. These are considerably cheaper while having high energy density, which means that they are smaller, weigh less and are more cost-effective. This makes them ideal choice for applications that require lower amount of energy as it helps cut down the cost as well as the weight of the final product.
Secondary cells are energy storage devices that can be reused multiple times. They can perform reversible cell reactions that allow them to recharge, by just reversing the direction of the current. Albeit being more expensive than the primary cells, they can be used multiple times, thus making them a better choice for energy storage applications that require a constant replenishment of energy, such as mobile phones, smart-watches, Electric vehicles, or large Battery Energy Storage systems for homes or discoms, etc.
There are a lot of different types of secondary cells available in the market, there are multiple variants of Lithium-ion cells, Nickel cells, Lead-acid batteries, hydrogen fuel cell, etc., all these batteries have their pros and cons. In this article, we will focus only on different chemistries of Li-ion Batteries.
Li-ion Cell
Lithium-ion cells are rechargeable cells, they use lithium as one of the key components in the construction of the cell. The development of Li-ion cells started in the early 70s, and their advancement and potential to catapult the energy storage systems making the adoption of EV’s a reality caused its inventors to win a Nobel Prize in Chemistry in 2019. These are rechargeable cells commonly used in portable electronics to something as large as Discoms for storing energy produced from renewable energy. There is a tremendous amount of research going on in the Li-ion batteries which cause the capacity of battery to increase drastically in the coming future. In the past 3 decades, the cost of battery has decreased by 97% and since the last decade, it has fallen by 88%.
Components of Li-ion Cell
Like any other cell, Li-ion cells are also made up of different components. These internal components help in shaping the features and other characteristics of a Li-ion cell. The Li-ion cell has 4 main components that are Cathode, Anode, Electrolyte, and Separator. The image below shows the different components of a Li-ion cell.
Cathode:
The material used in the cathode determines the capacity and voltage of Li-ion battery. This material is called the active material. The active material plays a crucial role in the chemical reaction in the battery which causes the flow of current. The capacity of a cell depends on the size of the active material at the cathode, i.e. for higher capacity of the cell, we need to have bigger cathodes. Meanwhile, the voltage of the cathode depends on the element used as a cathode.
The nomenclature of the battery usually depends on the cathode, like a Lithium-ion battery has a cathode made up of Lithium. But because lithium is highly reactive and unstable making it difficult to contain and use directly, therefore a combination of lithium and oxygen is used as a cathode.
Anode:
Similar to the cathode, an anode is the other electrode, which is also coated with an active material. It is the negative electrode and its property decides the rate of charge and discharge of the battery. We try to maximize the surface area of the anode which affects the rate of flow of electric current through the external circuit, resulting in a higher rate of absorption or emission of lithium ions released from the cathode. Anode stores the lithium ions during the charge cycle of the battery. The image below shows the discharging and charging states of the battery showing the flow of electrons and ions during the process.
The most commonly used anodes in Li-ion cells are graphite and the oxide spinel Li4Ti5O12. Graphene is being investigated and is considered a better alternative that can increase the power density as well as the charge and discharge rate of the cell.
Electrolyte:
An electrolyte solution is an electrically neutral solution, both the electrodes are submerged in the electrolyte solution. Although the solution is neutral but when an electric potential is applied, the ions start flowing towards the electrode. During the charge cycle, the lithium ions move from cathode to anode, while in the discharge cycle the reverse happens. Electrolyte plays a major role in the movement of ions thus making the flow of current possible. The movement of anions and cations in opposite directions in the electrolyte causes the flow of current.
For a Li-ion cell, the electrolyte needs to be a lithium salt, hence the most commonly used electrolyte in a Li-Ion battery is LiPF6 dissolved in an organic solvent.
Separator:
The separator plays an important role in the functioning of the battery. The electrolyte acts as a medium between the electrodes while the separator is placed between the electrodes to isolate them from each other so that no electron flows through them while allowing the ions to flow between them. The separator forms a catalyst when covered with the electrolyte thus facilitating the movement of ions between anode and cathode while stopping the electrons. When excessive heat is applied, the pores of separator get clogged by the melting process, this property can be employed as a safety feature, making the separator act as a fuse, stopping the flow of current in case of overheating thus safeguarding the battery from fire.
Most batteries for mobile phones and tablets which need to be kept lightweight and do not have a very rugged use have a single polyethylene separator while larger industrial batteries deploy tri-layered separator that acts like a fuse, protecting fires during extreme temperatures and on multi-cell configurations.
Types of Li-ion Battery
Li-ion batteries can be classified based on the combination of anode and cathodes used. There are six categories of lithium-ion battery readily available in the market, these are Lithium Cobalt Oxide (LCO), Lithium Manganese Oxide (LMO), Lithium Nickel Manganese Cobalt Oxide (NMO), Lithium Iron Phosphate (LFP), Lithium Nickel Cobalt Aluminum Oxide (NCA), and Lithium Titanate (LTO). Apart from the LTO battery, all other Li-ion battery has graphite as an anode. All the above Li-ion chemistries have different properties and we need to know their properties, their operating conditions and other parameters in order to select the best battery for our application. The properties of all the chemistries are given below.
1. LITHIUM COBALT OXIDE (LCO): Energy Dense but Low Thermal Stability
Lithium cobalt oxide battery have cobalt as the main active material in its cathode. It was invented in 1991 and has been extensively used because of its high energy density of 150-200 Wh/kg. Although this battery chemistry is energy dense, but other options are being investigated as the experts are claiming that the world could face a cobalt supply shortage soon. This is due to the surge in sales of electric vehicles where this battery chemistry is extensively used, leading to increase in cost over time.
Cobalt is a highly volatile metal, which limits the current handling capabilities of LCO battery due the risk of overheating. Furthermore, LCO batteries have lower thermal stability, that makes them sensitive to higher operating temperatures and overcharging.
LCO batteries are extensively used in portable electronics such as phones, cameras, laptops and have a high demand in electric vehicles.
2. LITHIUM MANGANESE OXIDE (LMO): The Safest Li-ion Chemistry
Lithium manganese oxide batteries are also known as lithium-ion manganese batteries. It has LiMn2O4 as a cathode. The earliest commercially developed battery with this chemistry was produced in 1996. These batteries have low internal resistance and high temperature stability which makes them safer than other lithium-ion battery types. LMO batteries are capable of delivering current up to 20-30 Amps due to their low internal resistance, thus making fast charging and discharging possible.
The main disadvantage of this chemistry is its relatively low cycle life of 300-700 cycles. These batteries also have lower capacity. Because of these drawbacks limited research and advancement of this battery type are expected in the future.
LMO batteries are extensively used in applications where high C-rates are required such as power tools. It also has applications in EVs and in medical applications.
3. LITHIUM IRON PHOSPHATE (LFP): Affordable, Safe, and Reliable
Lithium iron phosphate batteries use phosphate as active material in the cathode. These batteries are one of the most used chemistries in electric motorcycles, e-rikshaw as well as other applications that need a long lifecycle and significant safety. These batteries have a moderately high energy density of 90-160 Wh/kg. Similar to LMO, LFP chemistry have low internal resistance resulting in higher thermal stability. Unlike the LCO battery, these batteries are durable and have a long lifecycle with low self-discharge rates. LFP batteries have one of the best life cycles making them a very cost-effective option considering their long operations life. However, the nominal voltage of 3.2V of the li-phosphate battery means that it has less energy than other types of lithium batteries.
LFP batteries are used in industrial equipment and heavy machinery due to their high thermal stability and great life cycles. They are widely used in EVs, especially in e-bikes, e-rikshaw, and many cars because of their ability to withstand a lot of mechanical and thermal abuse.
4. LITHIUM NICKEL MANGANESE COBALT OXIDE (NMC): Expensive but High Performing Battery
Lithium Nickel Manganese Cobalt Oxide (NMC) Batteries uses a combination of nickel, manganese and cobalt as the active material for its cathode. The most commonly used ratio is 60 percent nickel mixed with 20 percent each of manganese and cobalt to form the alloy. Changing the ratio of these metals can alter the property and we can either attain a high specific energy density or a high specific power. These batteries are cheaper than other Li-ion batteries and have a very low self-heating rate and a nominal voltage of 3.7V. NMC batteries have energy density of 150-220 Wh/kg, which is higher than most other chemistries.
This battery is commonly used to power medical equipment, power tools and is considered as one of the preferred battery chemistries for EVs.
5. LITHIUM NICKEL COBALT ALUMINUM OXIDE (LINICOALO2) NCA: High Energy with Long Life
Lithium Nickle Cobalt batteries, also known as NCA batteries have a combination of nickel, cobalt and aluminum as active material in its cathode. These batteries have a high life cycle and are one of the most energy-dense Li-ion chemistry with energy density as high as 260Wh/kg and a nominal voltage of 3.6V. But the main disadvantage of this battery is its lower thermal stability and high cost making them an unviable option for consumer electronics. They are a good option for EVs due to their energy density, the NCA batteries must be used in cars with extra safety measures to monitor their performance and other data to keep the drivers secure.
The NCA batteries are becoming increasingly important in electric powertrains such as in Tesla and find application in grid storage due to their lifespan and energy density.
6. Lithium titanate LTO: Long life, fast charge using advanced Nanotechnology
Lithium titanate, also known as li-titanate are one of the newly developed Li-ion chemistries. They have advanced nanotechnology and replace the graphite used in the anode with lithium titanate as the active material. The large surface area of Li-titanate allows a larger quantity of electrons to enter and exit the anode faster, making a very high rate of charging and discharging possible without compromising on safety. The main drawback of this battery is that it suffers from is the low nominal voltage and low energy density of 2.4V and 50-80Wh/kg respectively. What makes this battery special is its ability to provide discharge rate exceeding 30 C for a short period of time. The use of advance nanotechnology makes it one of the safest chemistries available in today’s time.
Currently, a lot of big manufacturers of electric vehicles and bikes such as Mitsubishi, Honda, etc., use li-titanate batteries, and there is potential for this type of battery to be used in electric buses for public transportation.
LTO batteries have potential scope in aerospace, military and are used in battery energy storage systems for storing wind energy and solar energy and for creating smart grids. Their ability to sustain high discharge rates make them a preferred option for frequency control devices for grid applications.
Battery Comparison
The battery can be compared on many different parameters such as nominal voltage, the weight of the battery, specific energy, etc. The chart given below compares data of different chemistry of Li-ion cell. For reference, we have also added NiMh, Ni-cd battery in the table below.
Battery Chemistry | Temp min (℃) | Temp max (℃) | Cell Voltage (volts) | Self-discharge (% / month) | Cycles Times (max) | Weight |
NiCd | -20 | 60 | 1.2 | 20 | 800 | Heavy |
NiMH | -20 | 70 | 1.2 | 30 | 500 | Middle |
Low Self Discharge NiMH | -20 | 70 | 1.2 | 1 | 2000 | Middle |
Li-ion (LCO) | -40 | 70 | 3.6 | 10 | 1000 | Light |
Li-ion (LFP) | -40 | 80 | 3.2 | 5 | 12000 | Light |
LiPo (LCO) | -40 | 80 | 3.7 | 10 | 1000 | Lightest |
Li-Ti (LTO) | -40 | 55 | 2.4 | 5 | 20000 | Light |
LMO | - | 85 | 3.7 | - | 700 | Heavy |
For better representation of the above the data, the graphs have been drawn. The first graph represents the operating temperatures of different battery chemistry while the second graph shows the self-discharge rate of different battery chemistry.
From the above graph, it is clear that all the Li-ion chemistries have a broader operating range than the Nickel based batteries.
From the above graph showing the self-discharge rate, it is clear that most of the Li-ion cells have a very low self-discharge rate.
Comparison of Different Li-ion Chemistries
The property of Lithium-ion cell depends completely on the cell chemistry. All the chemistries have their own pros and cons which need to be considered while selecting a battery for a specific usage. The table listed below compares different Li-Ion chemistries.
Lithium Titanate (Li2TiO3) — LTO | Lithium Nickel Manganese Cobalt Oxide (LiNiMnCoO2) — NMC | Lithium Iron Phosphate(LiFePO4) — LFP | Lithium Nickel Cobalt Aluminum Oxide (LiNiCoAlO2) — NCA | Lithium Cobalt Oxide(LiCoO2) — LCO | |
Voltages Nominal (Volt) | 2.40V | 3.70V | 3.30V | 3.70V | 3.70V |
Typical operating range (V/cell) | 1.8V–2.85V | 3.0V–4.2V | 2.5V–3.65V | 3.0 V–4.2V | 3.0 V–4.2V |
Specific energy (Wh/kg) | 50–80Wh/kg | 150–220Wh/kg | 90–120Wh/kg | 200-260Wh/kg | 150–200Wh/kg. |
Charge (C-rate) | 1C typical; 5C maximum, charges to 2.85V | 0.7–1C, charges to 4.20V | 1C typical, charges to 3.65V | 0.7C, charges to 4.20V, fast charging possible with some cells | 0.7–1C, charges to 4.20V |
Discharge (C-rate) | 10C possible, 30C 5s pulse | 1C, 2C possible on some cells | 1C, 25C on some cells | 1C | 1C |
Cycle life | 3,000–7,000 | 1000–2000 | 5000-7000; up to 12000 possible in some cells | 500 | 500–1000 |
Thermal runaway | 200°C + | 210°C (410°F) | 270°C (518°F) | 150°C (302°F) | 150°C (302°F) |
Cost (per kWh) | ~$1,005 | ~$420 per | ~$580 | ~$350 | - |
Application | Electric vehicles, UPS, etc | E-bikes, E-Rikshaw, industrial equipment, etc | E-bikes, E-Rikshaw, Battery Energy storage system for discoms, offices and homes | Medical devices, industrial equipment, electric vehicles | Smart watches, mobile phones, tablets, laptops, cameras |
The graph shown below compares the nominal voltages of different Li-ion Chemistry.
From the above graph, we can say that the nominal voltage of NMC, LCO and LMO is higher than other chemistries while that of LTO is lowest. If we compare the data for energy density from the table, we find that the chemistry follows the same trend with LTO having the lowest energy density.
In terms of cycle life, LFP and LTO have the best cycle life while the NCA and LTO have a very low cycle life making them unfit for EVs.
LFP, NMC and LTO have the best thermal runaway making them most suitable for operations in extreme temperature.
When considering the costs, LTO performs well in most parameters, but the cost is not justifiable for consumer products and everyday portable equipment, whereas, NMC and LFP provides best of both worlds, they are competitively priced, while providing a decent performance and although NCA is cheaper than the other options but its poor thermal performance makes it dangerous for EVs and other operations where high temperature and mechanical stress is common, thus extra cost has to be considered for employing additional safety features to make it safe to use.
To sum up, it can be said that Li-ion batteries are the future of energy storage, the specification of cell depends on the internal composition, i.e. the material used for the electrodes, separator and the electrolyte. By changing the cathode, properties such as specific energy, charging rates, cycle life, nominal voltage, etc. can be controlled but every chemistry has their own pros and cons which needs to be considered while selecting the li-ion cell for a product.
In terms of cycle life, LFP and LTO have the best cycle life while the NCA and LTO have a very low cycle life making them unfit for EVs. LFP, NMC and LTO have the best thermal runaway making them most suitable for operations in extreme temperature. When considering the costs, LTO performs well in most parameters, but the cost is not justifiable for consumer products and everyday portable equipment, whereas, NMC and LFP provides best of both worlds, they are competitively priced, while providing a decent performance and although NCA is cheaper than the other options but its poor thermal performance makes it dangerous for EVs and other operations where high temperature and mechanical stress is common, thus extra cost has to be considered for employing additional safety features to make it safe to use.
A lot of research is going on in the field of battery and according to industry experts, the energy density of battery will increase with the cost expected to decline in the coming future.